Conserving glass artifacts at Jamestown
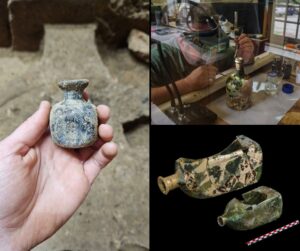
April 30, 2025. Dr. Chris Wilkins, Senior Conservator.
The general role of an archaeological conservator is to stop degradation and corrosion of artifacts as archaeologists unearth them. It is crucial for conservators to have an understanding of the degradation and corrosion process of various types of materials. In this sense, a conservator is also a materials scientist who focuses on historical materials.
At Jamestown Rediscovery, we’ve been focusing on glass objects recovered from our excavations. This focus is the result of conservation of recently-excavated glass from Jamestown, conservation of several glass bottles recovered from excavations at George Washington’s Mount Vernon, and the ongoing care and management of the Jamestown glass collections (see “In conservation, this will probably be the hardest thing you ever do.” | Historic Jamestowne; Fig. 1).
To conserve a glass artifact, a conservator needs to know the process of glass production, its general history, and glass degradation/decomposition. Additionally, the conservator and responsible party (e.g. curator, stakeholder, and/or owner) consult in the proper storage of glass based on its recovery and condition. There are different types of glasses, let us explore one.
Glass Production
Did you know?
Myth: Glass Flows
Glass is a supercooled liquid due to its non-uniform molecular structure. Glass does not flow and recent analysis of 20 million year old amber has proven this. Glass panes are thicker at the bottom in old windows because it was difficult to produce plate glass with an even thickness in the past, and those who constructed the windows thought the glass panes were more stable with the heavier thick side down.
Alkali glasses are the most abundant glass type throughout history. The production of these glasses requires at least three components: silicon, alkali (sodium and/or potassium), and calcium. Glass is actually mostly silica, so why do we need these other elements?
Melting pure silica sand will produce a stable glass called fused silica glass. However, the melting point of silica is 1713°C (3115°F), a difficult temperature to reach in both kilns from the past and most kilns today.
An alkali fluxing agent is required to reduce the melting point to a more kiln-acceptable level of 800-1000°C (1472-1832°F) for softening or 1400-1600°C (2552-2912°F) for a true melt. The fluxing agent also lowers the viscosity of the glass melt, making it easier to work and form. Glasses made with fluxing agents, like sodium, are known as alkali glass. However, pure silica-sodium glass is unstable and will quickly dissolve in water or become crizzled (intact highly fractured glass) over time. The introduction of calcium oxide to the mixture counters this by stabilizing the glass, if included in appropriate amounts. Adding other materials to the glass mixture allows for manipulation of opacity and color.
A Brief History of Glass
Did you know?
When adding the alkali components together (Na2O + K2O), a comparison of Roman glass from across the Empire and 17th C. English glass revealed a very similar makeup despite a separation of 1600 years.
Components | Roman | 17th C English |
SiO2 | 65-74% | 65.40% |
Na2O | 11-19% | 6.60% |
K2O | 0.3-1.5% | 11.80% |
CaO | 4.6-10% | 6.50% |
Na2O + K2O | 11.03-20.5% | 18.40% |
Pliny the Elder (d. A.D. 79 at Pompeii) recounts a story of the discovery of glass by natron traders landing on the shores near the colony of Ptolemais at the mouth of the River Belus in present-day Israel (Natural History XXXVI:192). Not finding any stones to support their cookware, they used natron blocks from their stores and, under the heat of the fire, a molten glass formed beneath. Reflecting on the components of a glass mentioned in the preceding paragraph, upon the sandy, presumably shelly, beaches, this mixture of sand (silica), natron (sodium), wood ash (sodium/potassium) and shell (calcium), could have produced an unintended glass (Gorem-Rosen 2000).
However, the manufacture of glass certainly predates Pliny, with evidence of it having occurred as early as 3500 BCE in the Near East (Pfeander 1996) if not earlier in other places like Mycenae, China, and North Tyrol. A clay tablet recovered from the library of the Assyrian King Ashurbanipal (696-626 BCE) provides a glass recipe and states that glass can be produced from ‘60 parts sand, 180 parts ashes of sea plants, 5 parts chalke’ equating to 25% sand (silica), 74% ash (sodium oxide and potassium oxide) and 2% chalk (calcium oxide) (tablet | British Museum). While the ingredients for glass (silica, sodium/potassium, calcium respectively) are good, the quantities provided would not produce a good quality glass.
Glass is a conservative technology with very little change over long periods. Analysis of clear and colored Roman glass from across the empire revealed compositions of largely silica (SiO2), with some sodium, potassium and calcium oxides (Na2O, K2O and CaO), with additional inclusions for color, opacity and incidentals (Ganio et al. 2012 ). The composition of typical 17th-century English alkali glass is similar (Dungworth and Brain 2009). Totaling the alkalis reveals little significant difference between Roman and English glass despite a separation of 1600 years!
Did you know?
Crystal Glassware (Lead Glass)
Crystal is also known as ‘lead glass’ and ‘lead crystal’. Its name is derived from the Venetian word cristallo, meaning ‘like rock crystal’. Lead glass is similar to alkali glass but uses lead as a fluxing agent replacing most of the sodium and potassium, further reducing the working temperature and viscosity of the melt. The result is a higher refractive glass with greater brilliance and sparkle than alkali glass. It degrades in a similar process to alkali glass but the leaching of lead can leave white lead salts on the surface near the affected area.
Glass Degradation
The degradation of glass is dependent on its composition and the environment. Modern fused silica glass is an extremely stable material. Most archaeological glass, however, is alkali glass. Alkali glass can become crizzled and could eventually fall apart if the amount of calcium originally added to the mixture during production is not appropriate for glass stabilization, although this is more common with historical glass than archaeological glass.
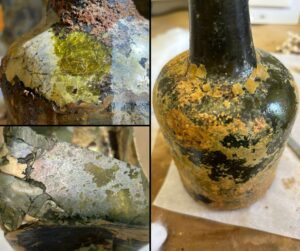
Archaeological glass is susceptible to two competing degradation processes involving water: dealkalization and network dissolution (Freestone 2001). Dealkalization simply means removing alkalis (sodium or potassium). Water will leach the alkali and some calcium from the glass structure replacing it with smaller hydrogen atoms from the water. The replacement of larger atoms with smaller atoms forces the contraction of the affected layers. This contraction causes the layers to partially separate from the pristine glass below and eventually flake off (Fig. 2). Known as ‘gel’ layers, they will expand and contract depending on the presence of moisture much like a true gel. Each layer, as seen by the naked eye, is actually several layers. Refraction of light passing through them can make them appear iridescent or avariation of dull opaque browns. Loss of these layers results in a loss of the original dimensionality of the object as well as any inscriptions and adornments connected to that surface.
Network dissolution is the removal of silica, the main component of glass. Ground water generally has a pH of 7. Dealkalization leaches sodium from the glass into the surrounding ground water causing the pH to increase.
Did you know?
‘Soap residue’ on your glassware that consistently reappears when you dry the glass is probably an indication that the glass is in the early stages of dealkalization.
If the pH goes above 9.5, network dissolution becomes dominant over dealkalization. For instance, if rainfall rinses away the water, the pH never becomes critical. If stagnant, the higher-pH water causes network dissolution, or the loss of silica, which results in pitted glass and/or general surface erosion and a generally weaker glass object. A glass object where significant network dissolution has occurred may appear intact in-situ but can fall apart upon simple handling.
Glass Conservation
The condition of the glass determines the conservation that has to occur to save the object. If glass is wet, or ‘waterlogged’, and has gel layers due to dealkalization, it has to be control-dried to ensure the gel layers do not flake away from the object. Placing the glass into successive water baths with increasing concentrations of ethanol and letting the glass dry afterwards will result in less loss.
Glass recovered from Jamestown is not usually waterlogged but does have soil ‘adhered’ to it. Uncontrolled removal of the dirt by a conservator can pull away the gel layers or even larger fragments of glass revealing pristine glass located a few millimeters below the original surface. While ‘pristine glass’ may sound ideal, removal of the gel layers results in the loss of anything that was on the original glass surface, like inscriptions, as well as a loss in dimensionality. A dilute adhesive applied to the adhered soil and glass locks the gel layers into place and allows the conservator to control the removal of dirt from the surface. At Jamestown, we use a mixture of B-72 and acetone. Using a scalpel and/or solvent-impregnated cotton buds, mechanical cleaning occurs centimeter-by-centimeter across the surface to expose the glass and gel layers (Fig 3, also see Fig. 1).
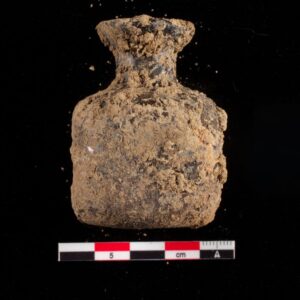
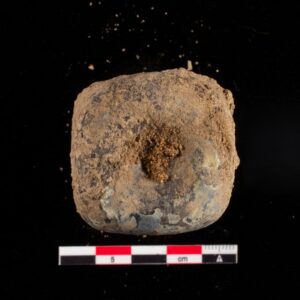
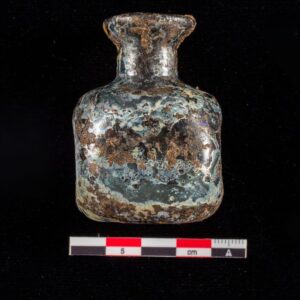
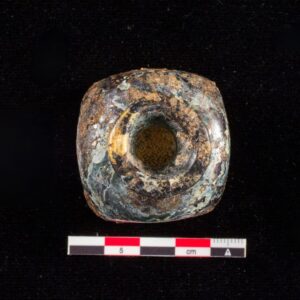
Once the glass is cleaned, application of a final consolidation layer in the form of a dilute adhesive produces a glassy finish while protecting the gel layers. Adhesive between the gel layers decreases light refraction, causing the colors on the surface to appear muted.
Glass Storage
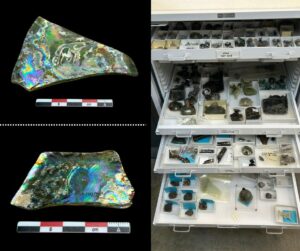
The condition of the glass when excavated also determines the way it should be stored. Glass found in the desert or as a part of an historic collection is best placed in a dry environment. This kind of glass can survive in very low relative humidity. Glass recovered from archaeological excavations in wetter environments, like Jamestown, could still have molecule-thick layers of water in crevices and between gel layers, even if the glass appears dry. Placing this glass into a dry storage location could cause the gel layers to flake off as the water evaporates. A relative humidity of 45-50% (as found in your typical household) is ideal for archaeological glass. At a higher relative humidity, moisture could collect on the glass surface restarting the dealkalization process and further degrading the object.
Mechanical damage is an immediate concern when handling glass, archaeological or otherwise. Knocking a cannonball off a worktable will make a large sound, and at worse, break a toe. Dropping a glass object from the same height will likely cause it to shatter with potential loss of shards and possible cuts to the person trying to retrieve it quickly. Placing the glass into housings (e.g. bag or box) while in storage helps to protect the object from mechanical damage. If the box is dropped and the object breaks, the shards are contained with no loss and can be handled when time allows. A simple labeled box, with supports for the object inside, placed securely on the storage shelf and tracked through a database ensures a higher probability that the object will remain intact and available for the future. Boxes can be dismissed if specialized cabinets are used. These usually have multiple removable, adjustable shelves and, sometimes, a glass front but still serve to protect the contents within from mechanical damage (Fig. 4) and buffers changes in temperature and relative humidity.
In Conclusion
Next time you have a sip of iced tea or hot coffee from a glass, think of the 5000 years of glass history and innovation that went into it. Think of how we, as humans, thought to crush sand and shell, and combine it with ashes of wood and plants, and/or natural salt deposits to produce a material that can be transparent or opaque and any number of colors. A material that is both strong yet fragile, but where simple exposure to water coupled with an extent of time can pull it apart atom by atom. And think of us, preserving this glass against the will of physics and nature so that those in the future can learn about and see the beauty of the past.
References Cited:
Dungworth, D. and Brain, C. (2009). Late 17th–Century Crystal Glass: An Analytical Investigation. Journal of Glass Studies 51
Freestone, I. (2001). Post-Depositional Changes in Archaeological Ceramics and Glass. Handbook of Archaeological Sciences. D. R. Brothwell and A. M. Pollard, John Wiley & Sons: 615-625
Ganio, M., S. Boyen, T. Fenn, R. Scott, S. Vanhoutte, D. Gimingo and P. Degryse (2012). Roman Glass Across the Empire: An Elemental and Isotopic Characterization. Journal of Analytical Atomic Spectrometry 5
Gorin-Rosen Y. (2000). The Ancient Glass Industry in Isreal, Summary of the Find and New Discoveries. In M.D. Nenna, La route du verre: Ateliers primaires at secondairs du second millénaire av. J.-C. au Moyen Âge (Travaux de la maison de l’Orient Méditeranéen 33). Lyon. Pp. 49-63
Pfaender, H.G. (1996). The History of Glass. Schott Guide to Glass. Dordrecht, Springer Netherlands: 1-15